Atom: What it is, structure and applications today
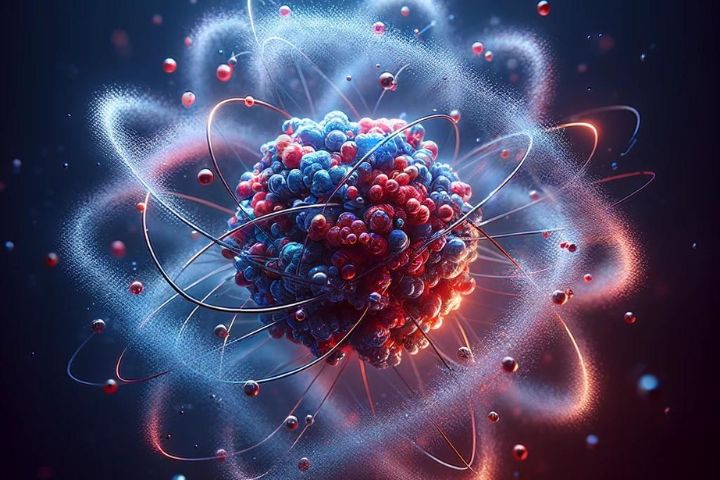
The atom, from its philosophical conception in ancient times to its current understanding in modern physics, has been the fundamental pillar on which our understanding of the physical universe is built.
Today we will talk about
- 1. History of the concept of the atom: From philosophy to quantum physics
- 2. Quantum mechanics and the current atom model
- 3. Isotopes and radioactivity
- 4. Principles of quantum mechanics applied to the atom
- 5. Formation of molecules: Bonds and structures
- 6. The atom and fundamental forces
- 7. Spectroscopy and atoms
- 8. Nuclear fission and fusion
- 9. Final summary of the article on the atom
- 10. Recommended readings on the atom
Constituting the basic unit of matter, the atom is composed of a central, positively charged nucleus, surrounded by a cloud of negatively charged electrons. This structure, although seemingly simple, encapsulates the complexities inherent in nature's fundamental interactions.
History of the concept of the atom: From philosophy to quantum physics
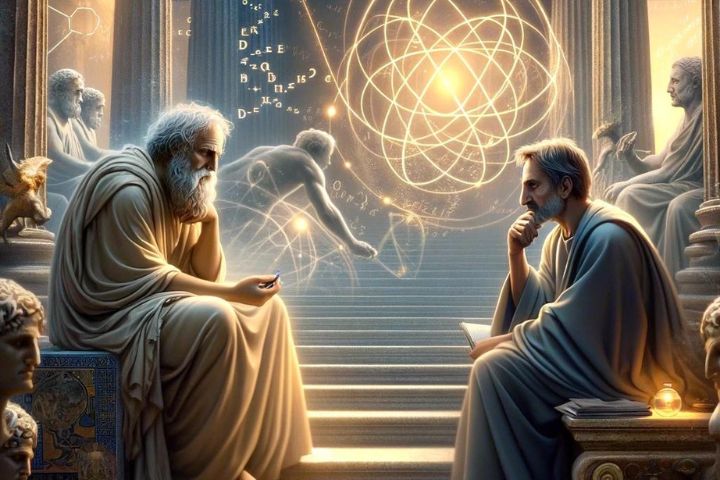
The history of the concept of the atom is a fascinating journey through time, from its philosophical origins in ancient Greece to the complexities of modern quantum physics. This journey takes us from the first speculations about the indivisible nature of matter to the discovery of a subatomic reality that challenges our intuition and traditional understanding of the world.
Over the centuries, the atom has been the protagonist of constant debate, evolving through the contributions of thinkers and scientists who have unraveled the mysteries of matter and the forces that govern it. The transition from philosophy to quantum physics reflects not only the advancement of human knowledge but also the change in our way of interrogating and understanding the universe at a fundamental level.
Ancient philosophical ideas about the atom
The notion of the atom dates back to ancient Greece, where philosophers such as Democritus and Leucippus speculated on the existence of indivisible and invisible particles as the fundamental constituents of matter. Although his ideas lacked experimental evidence, they established the conceptual basis for future scientific exploration of the atom.
Dalton's atomic model
In the 19th century, John Dalton revitalized the concept of the atom with his atomic theory, proposing that each element is composed of atoms of the same mass and properties, thus differentiating them from each other. This theory, based on chemical experiments, marked the beginning of modern atomic science.
The discovery of the electron and Thomson's model
The discovery of the electron by J.J. Thomson in 1897, through his experiments with cathode ray tubes, revealed that the atom had a complex internal structure. This led to the development of his "plum pudding" model, where electrons were embedded in a positively charged sphere.
Rutherford's experiment and the atomic nucleus
Alpha particle scattering experiments conducted by Ernest Rutherford in 1911 provided the first evidence of a heavy, concentrated atomic nucleus, challenging Thomson's model. This led to the planetary model of the atom, where electrons orbit the central nucleus.
Bohr's atomic model
Niels Bohr integrated quantum into the atomic model in 1913, introducing stable orbits for electrons and explaining the emission of specific light spectra by atoms. His work laid the foundation for quantum mechanics.
Quantum mechanics and the current model of the atom
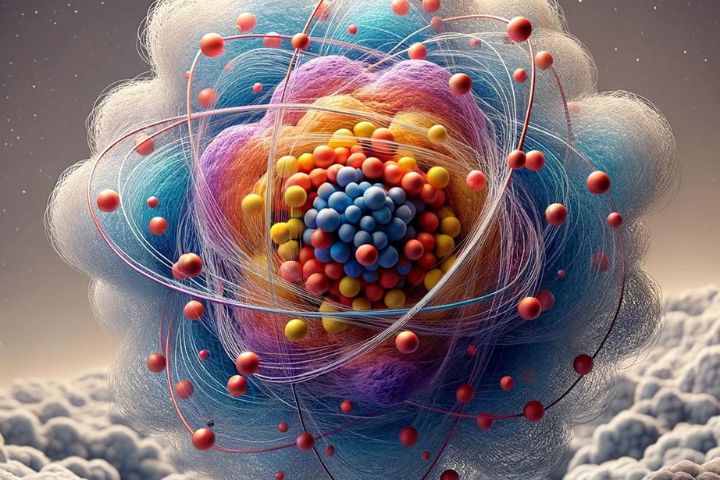
Quantum mechanics, developed in the 1920s and 1930s by scientists such as Schrödinger, Heisenberg, and Dirac, revolutionized our understanding of the atom. The current model It describes electrons in terms of probabilities, occupying "orbitals" rather than defined orbits, and is governed by the Heisenberg uncertainty principle.
Atomic structure: the nucleus and the electronic cloud
The modern understanding of atomic structure is based on the duality of its main components: the nucleus, composed of protons and neutrons, and the electron cloud, which houses the electrons. The densely packed, positively charged nucleus contains most of the atom's mass, while the electrons, with their negative charge, define the size and shape of the atom.
Atomic nucleus: Protons and neutrons
The atomic nucleus is characterized by incredible density and strong stability, thanks to the strong nuclear force that holds protons and neutrons together. This force, operative only at extremely short distances, is responsible for counteracting the electromagnetic repulsion between positively charged protons.
The electronic cloud: Electrons
Electrons, on the other hand, occupy regions of space around the nucleus known as atomic orbitals, areas where the probability of finding an electron is greatest. The distribution and energy of these electrons determine the chemical properties of the elements, as well as their interactions with other atoms.
Isotopes and radioactivity
Definition of isotopes
Isotopes are variants of the same element that have the same number of protons but a different number of neutrons in their nuclei. This variation in the number of neutrons affects the mass of the atom but not its chemical properties. Isotopes have crucial applications in fields such as medicine, archeology and nuclear energy.
Radioactivity concept
Radioactivity is a process by which unstable nuclei emit particles or electromagnetic radiation to reach a more stable state. This natural phenomenon is fundamental to understanding processes such as nuclear fusion in stars and radiometric dating of artifacts and fossils.
Principles of quantum mechanics applied to the atom
Quantum mechanics, with its non-intuitive principles, is essential to describe the behavior of electrons in the atom.
Wave-particle duality
This principle states that subatomic particles, such as electrons, can exhibit properties of both particles and waves, depending on the experiment performed. This duality is crucial to understanding phenomena such as electron diffraction and the formation of interference patterns.
Heisenberg uncertainty principle
The uncertainty principle proposes that it is impossible to determine simultaneously and with absolute precision the position and velocity of a particle. In the context of the atom, this means that we cannot exactly know the position of an electron and its momentum (mass times velocity) at the same time, leading to a probabilistic description of its position in the form of atomic orbitals.
Atomic orbitals and quantum numbers
Atomic orbitals are regions of space around the nucleus where the probability of finding an electron is high. Quantum numbers describe the properties of these electrons, including their energy, angular momentum, and spatial orientation. The electronic configuration, determined by the distribution of electrons in orbitals, is key to understanding the chemistry of elements.
Formation of molecules: Bonds and structures
Atoms do not exist in isolation in nature; Their tendency to combine and form molecules is fundamental to chemistry and biology. This section will explore the different types of atomic bonds and how they lead to the formation of molecules with specific properties.
Covalent bond: sharing electrons
Covalent bonding is formed when two atoms share one or more pairs of electrons, stabilizing their electronic configurations. This type of bond is key to the formation of organic molecules, including those essential for life, such as DNA and proteins.
Ionic bond: electron transfer
In ionic bonding, one atom donates electrons to another, resulting in the formation of ions with opposite charges that attract each other. This type of bond is common in inorganic compounds, such as salts.
Metallic bond: sea of electrons
Metallic bonding, characteristic of metals, occurs when electrons move freely between a network of positive ions. This behavior explains metallic properties such as electrical and thermal conductivity.
The atom and fundamental forces
Understanding the atom and its behavior under different fundamental forces is essential to physics. These forces are responsible for the interaction between the basic components of the atom and between the atoms themselves.
Electromagnetic interaction
Electromagnetic interaction is one of the four fundamental forces of nature and is crucial for the structure and stability of atoms. This force acts between electrically charged particles, such as protons and electrons, and is responsible for the attraction between electrons (negatively charged) and atomic nuclei (positively charged).
Electromagnetic interaction not only keeps electrons in orbits around the nucleus, but is also fundamental in the formation of chemical bonds between atoms, allowing the existence of molecules and, by extension, all matter in the state we know.
Strong and weak nuclear force
The strong nuclear force is another of the fundamental forces and is what allows the cohesion of the atomic nucleus, keeping the protons and neutrons together despite the electromagnetic repulsion between the protons. This force acts only at very short distances, on the order of femtometers (10^-15 meters), but is extremely powerful, far surpassing the electromagnetic force at that scale. Without the strong nuclear force, atomic nuclei could not exist.
The weak nuclear force, on the other hand, is responsible for certain types of nuclear decay and nuclear reactions that change one type of subatomic particle into another, as in the case of beta decay. Although weak compared to the other nuclear forces, the weak nuclear force plays a crucial role in nuclear processes and radioactivity.
Gravitation on the atomic scale
Although gravitation is the most familiar force in our everyday experience, it is the weakest of the four fundamental forces, especially at the atomic scale. Its effect is negligible on the behavior of electrons around the nucleus. However, at massive scales, such as planets, stars, and galaxies, gravitation dominates the structure and dynamics of the universe.
Spectroscopy and atoms
Spectroscopy is a powerful analytical technique that allows the identification of elements and compounds through the analysis of the light emitted or absorbed by them.
Fundamentals of spectroscopy
Spectroscopy is based on the principle that atoms and molecules can absorb or emit light (electromagnetic radiation) at specific wavelengths, resulting in a unique spectrum for each element or compound. This phenomenon occurs because electrons in an atom can move between different energy levels, absorbing or emitting photons with specific energies (and therefore wavelengths) in the process.
Applications of spectroscopy in the study of atoms
Spectroscopy is fundamental in atomic physics to study the structure and properties of atoms. It allows us to determine the composition of materials and substances, identify elements in unknown samples, and study the physical conditions of distant systems, such as stars and galaxies.
Identification of elements through spectra
Each element has a unique spectrum, a "fingerprint" that allows its precise identification. This is especially useful in astrophysics, where spectroscopy helps determine the chemical composition of stars and galaxies based on the light reaching Earth from these celestial objects.
Nuclear fission and fusion
These nuclear processes are fundamental in both energy generation and stellar reactions.
Nuclear fission is the process of splitting a heavy nucleus into two or more lighter nuclei, releasing a large amount of energy. Nuclear fusion, on the other hand, combines light nuclei to form a heavier one, releasing even greater energy. Both processes are possible due to differences in nuclear binding energy per nucleon between the nuclei involved.
Applications: nuclear energy
Nuclear fission is the basis of nuclear energy used in nuclear reactors to generate electricity. It is also the principle behind nuclear weapons. Nuclear fusion, although it has not yet been achieved in a sustained and controlled manner on Earth for energy generation purposes, is the process that powers the Sun and other stars, being investigated as a potential inexhaustible source of clean energy.
Stars and nuclear fusion
Stars, including our Sun, are gigantic nuclear fusion reactors. The fusion of hydrogen into helium in their nuclei releases the energy that sustains stellar radiation and, therefore, life on Earth. Understanding nuclear fusion not only has implications for energy generation but also for understanding stellar evolution and the formation of elements in the universe.
Final summary of the article on the atom
The atom, despite its invisibility to the naked eye, is the foundation of all matter and the basis on which the physical universe is built. From the formation of stars and planets to the diversity of life on Earth, it all comes down to how atoms interact with each other.
Atomic physics has transformed our understanding of the world, enabling technological developments ranging from nuclear power generation to nanotechnology and modern medicine. The ability to manipulate atoms and molecules has led to innovations in electronics, materials, and beyond, shaping contemporary society in ways we are only beginning to understand.
Looking to the future, atomic research continues to push the limits of our knowledge and technological capability. With challenges such as dark matter, dark energy, and the unification of fundamental forces, the field is far from having reached its limits.
Furthermore, the promise of new technologies, such as quantum computing and nuclear fusion, points to a future in which complete mastery over the atom could once again transform the way we live and understand the cosmos. As we move forward, human curiosity and the desire to explore the unknown will continue to be the spark that propels atomic research toward new horizons.
Recommended readings on the atom
For those interested in delving even further into the fascinating world of the atom and its impact on physics, chemistry and technology, here is a selection of further reading. These books range from the history and development of the concept of the atom to the most recent advances in atomic and quantum physics, offering readers a deep and nuanced understanding of this fundamental component of the universe.
- "The Atom in the History of Human Thought" by Bernard Pullman. This book offers a comprehensive historical exploration of the concept of the atom from ancient times to the 20th century. Pullman details how ideas about the atom have evolved and how they have influenced human thinking throughout history.
- "Atom: Journey Across the Subatomic Cosmos" by Isaac Asimov. Isaac Asimov, in his characteristically clear and accessible style, guides readers through the world of atomic physics, from the earliest philosophical concepts of matter to the complex principles of quantum mechanics.
- "The Quantum Universe: (And Why Anything That Can Happen, Does)" by Brian Cox and Jeff Forshaw. In this book, Cox and Forshaw unravel the principles of quantum mechanics and their application to the study of atoms. It is a fascinating read for those seeking to understand how quantum laws govern the behavior of matter at subatomic scales.
- "Quantum: A Guide for the Perplexed" by Jim Al-Khalili. Jim Al-Khalili presents a comprehensive introduction to quantum theory and explores its application in the atomic world. This book is ideal for readers who want a clear explanation of complex concepts without an overload of technical jargon.
- "The Making of the Atomic Bomb" by Richard Rhodes. Winner of the Pulitzer Prize, this book tells the fascinating and often disturbing story of the development of the atomic bomb. Although it focuses on a specific aspect of atomic physics, it provides important context on the ethical and social implications of atomic science.
- "Nuclear and Particle Physics: An Introduction" by Brian R. Martin. This introductory text is perfect for students and readers with a serious interest in nuclear and particle physics. Martin covers the fundamentals of both fields with clarity and depth, making complex concepts accessible.