Kinetic Energy: Definition, Formula, History, Applications and Solved Exercises
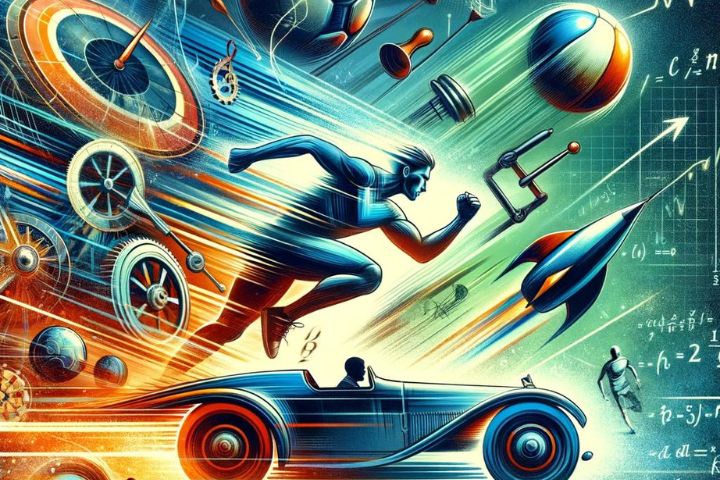
Today we will talk about
- 1. What is Kinetic Energy?
- 2. Fundamental characteristics of kinetic energy
- 3. Derivation of the kinetic energy formula
- 4. Everyday examples of kinetic energy
- 5. Types of Kinetic Energy
- 6. Kinetic energy in relativistic mechanics
- 7. History of kinetic energy
- 8. Problems and exercises solved on kinetic energy
What is Kinetic Energy?
Kinetic energy, a fundamental concept in physics, is the energy that a body possesses due to its movement.
This concept, which lies at the heart of classical mechanics, is essential for understanding a wide range of natural and technological phenomena, from the movement of the planets in the solar system to the most innovative applications in engineering and technology.
Exploring kinetic energy not only allows us to describe and predict the behavior of moving objects accurately, but also gives us a deeper understanding of the laws that govern the universe.
The history of kinetic energy dates back to the dawn of science, evolving through the contributions of ancient philosophers, astronomers, and physicists, to contemporary developments in classical mechanics, relativity, and quantum mechanics. This concept plays a crucial role in the principle of conservation of energy, one of the fundamental laws of physics, which states that energy is neither created nor destroyed, but rather transformed from one form to another.
The study of kinetic energy is not only relevant to theoretical physics, but also has significant practical applications. In engineering, for example, understanding how kinetic energy is transferred and transformed is essential to the design of more efficient machines and transportation systems.
In the field of biology, it helps explain the mechanisms behind the movement of living beings, from human locomotion to bird flight.
This article aims to offer a comprehensive vision of kinetic energy, addressing its theoretical foundations, its historical development, and its application in various fields of study.
Through a clear and detailed presentation, we seek to provide a deep understanding of this vital concept, accessible to both science students and professionals, and to anyone interested in the wonders of physics and mathematics.
Fundamental characteristics of kinetic energy
- Dependence of Mass and Velocity: The amount of kinetic energy that an object has depends directly on its mass and the square of its speed. This means that a heavier or faster moving object will have more kinetic energy.
- Scalar Energy: Unlike velocity, which is a vector quantity and has direction, kinetic energy is a scalar quantity. This means that it only has magnitude and no direction.
- Energy Transformation: Kinetic energy can be transformed into other forms of energy, such as potential energy, and vice versa, but the total amount of energy in a closed system is conserved. This principle is known as the conservation of energy.
Derivation of the kinetic energy formula
The equation we use to calculate the kinetic energy of an object is the result of experimental observations and fundamental mathematical analyzes in physics. The formula:
Ec = 1/2mv^2
It is derived by considering the work done on an object to move it from one position to another. Here, m is the mass of the object and v It's your speed. The ½ arises from the integration of the work done against the constant force.
Importance of the square of the velocity in kinetic energy
The fact that kinetic energy depends on the square of velocity has profound implications. It means that a small increase in the speed of an object can result in a large increase in its kinetic energy. For example, if an object's speed doubles, its kinetic energy quadruples.
Everyday examples of kinetic energy

Kinetic energy, being a form of energy associated with movement, manifests itself in countless everyday situations. These examples illustrate how kinetic energy is present in our daily lives, often in ways we take for granted:
- Walk or Run: When we walk or run, our body is in motion, possessing kinetic energy. The faster we move, the greater the kinetic energy we generate.
- Moving Vehicles: Cars, bicycles, motorcycles, and other vehicles have kinetic energy when they move. This energy increases with the mass of the vehicle and the square of its speed, which explains why a higher speed impact can be much more destructive.
- Balls in Play: A ball thrown, kicked or hit during games or sports has kinetic energy. The speed of the ball and its mass determine how much energy it has. When you catch it, that kinetic energy is dissipated, usually in the form of heat and work done on the ball to stop it.
- Celestial bodies: Planets moving around the Sun, the Moon orbiting the Earth, and artificial satellites around the Earth all possess kinetic energy due to their orbital or rotational motions.
- Flowing Water: The water that flows in rivers, waterfalls, or even from the faucet in our house, has kinetic energy. Hydroelectric power harnesses this form of kinetic energy, converting it into electricity.
- Windmills and Wind Turbines: These devices convert the kinetic energy of the wind into mechanical energy (and, in the case of wind turbines, often electrical energy). The amount of kinetic energy captured depends on the wind speed and the mass of air passing through the blades.
- Mobile Toys: Toys that move, such as toy cars, trains, or airplanes, have kinetic energy. This energy is transferred to the toys from our hand (when pushing them) or from batteries (in the case of electric toys).
- Recreational activities: Skateboarding, skiing, ice skating, or sliding down a slide are activities where kinetic energy plays a crucial role. As speed increases in these activities, so does the kinetic energy involved.
- Free Fall of Objects: When we drop an object, it gains kinetic energy as it accelerates toward the ground, due to gravity. Gravitational potential energy is converted to kinetic energy during the fall.
Types of Kinetic Energy
Kinetic energy comes in various forms, depending on the nature of the objects' motion. These forms can be classified into two main categories: translational kinetic energy and rotational kinetic energy. Below, we will explore each of these forms, providing a deeper understanding of how kinetic energy manifests in different contexts.
Translational Kinetic Energy
Translational kinetic energy is the energy that an object possesses due to its translational motion, that is, its movement from one place to another. This type of kinetic energy depends on the mass of the object and the square of its speed, according to the relationship:
Ek= 1/2mv^2
where Ek is the kinetic energy, m is the mass of the object and v It's your speed.
Examples:
- A car traveling on a road.
- A ball that is thrown or hit.
- A person running.
Rotational Kinetic Energy
Rotational kinetic energy is the energy that an object possesses due to its rotation around an axis. This type of energy depends on the moment of inertia of the object and the square of its angular velocity, and is calculated by the formula:
Ek = 1/2Iω^2
where
Ek is the kinetic energy, Yo is the moment of inertia of the object with respect to the axis of rotation, and ω is the angular velocity.
Examples:
- A millstone turning.
- A rotating music disc or DVD.
- The Earth rotating on its axis.
Vibration Kinetic Energy
Although not a major category like the previous ones, vibrational kinetic energy is another important form, especially in the context of molecules. This energy is the result of the vibrational movement of atoms within a molecule.
Example:
- The vibrations of the molecules in a gas, liquid, or solid, which contribute to its internal thermal energy.
Interconnection between types of kinetic energy
It is crucial to understand that these types of kinetic energy do not exist in isolation; They are often combined or transformed from one to another. For example, an airplane in flight has translational kinetic energy due to its motion through the air, but its engines and moving parts, such as turbines, have rotational kinetic energy. Likewise, the air molecules flowing around and within the aircraft have kinetic energy of vibration, contributing to the thermal energy of the system.
These interactions underscore the complexity and pervasiveness of kinetic energy in the physical world, demonstrating how different forms of motion contribute to the total energy of systems in our daily lives and in the universe at large.
Kinetic energy in relativistic mechanics
The theory of special relativity, formulated by Albert Einstein, redefines our understanding of many fundamental concepts of physics when applied to contexts where objects move at extremely high speeds, close to the speed of light. This theory has profound implications for how we understand kinetic energy, which is the energy an object possesses due to its motion.
Fundamentals of Relativistic Mechanics
Within the framework of classical physics, we understand kinetic energy as the energy that an object acquires by moving. However, this explanation is based on speeds much less than the speed of light.
At speeds that are a significant fraction of the speed of light, special relativity theory shows that the rules change. According to this theory, as an object approaches the speed of light, its kinetic energy increases in a non-linear manner, that is, it does not follow the uniform increase that we would expect in low speed situations.
Implications of Relativistic Kinetic Energy
- Mass Increase: One of the most fascinating results of the theory of relativity is that, at high speeds, the mass of an object appears to increase. This means that the faster an object tries to move, the more energy is required to accelerate it, making reaching or exceeding the speed of light impossible for objects with mass.
- Energy Conversion: Einstein's famous equation, which relates mass and energy, suggests that the energy used to accelerate an object not only increases its speed but can also increase its mass. This has important consequences for how we understand the relationship between an object's mass and its energy.
- Practical applications: These principles of relativistic mechanics are crucial in fields such as particle accelerators, where particles move at speeds that are a significant fraction of the speed of light. Here, the relativistic kinetic energy of the particles is a critical factor in understanding how they interact and the reactions that occur as a result.
- Astrophysics and Cosmology: In the cosmos, where objects such as galaxies and black holes move at extremely high speeds, special relativity is essential to analyze and understand phenomena such as the relativistic jets of black holes or the movement and interaction of galaxies on large scales.
History of kinetic energy
The history of kinetic energy is a fascinating journey through time, taking us from the first observations and theories of motion to the development of fundamental concepts in modern physics. This evolution has not only deepened our understanding of the universe, but has also been instrumental in the development of advanced technologies and improving our quality of life.
The origins: natural philosophy and first theories
The history of kinetic energy begins in ancient times, with natural philosophers such as Aristotle, who proposed theories about motion and dynamics. However, these early theories often lacked the precision and mathematical rigor that would characterize later developments in physics.
The scientific revolution: Galileo and Newton
True understanding of motion and kinetic energy began to take shape during the Scientific Revolution, thanks to the works of Galileo Galilei and Sir Isaac Newton. Galileo challenged Aristotelian ideas and conducted experiments that showed that the speed of objects in free fall increases uniformly, a concept that laid the foundation for understanding the relationship between motion and energy.
Sir Isaac Newton, for his part, formulated the laws of motion that bear his name, establishing the fundamental principles of classical mechanics. Although the term "kinetic energy" was not yet used, its laws made it possible to mathematically describe the motion and dynamics of objects, including the concepts that would eventually be recognized as kinetic energy.
The 19th century: Development of the concept of energy
The concept of energy, including kinetic energy as we know it today, was developed more formally in the 19th century. It was in this period that scientists began to understand energy as a conserved quantity that can be transformed from one form to another but not destroyed. This principle of conservation of energy was crucial to the development of thermodynamics and statistical mechanics.
Kinetic energy, specifically, was defined and used in the analysis of machines and in the description of mechanical work. Engineers and scientists such as Gaspard-Gustave de Coriolis and Jean-Victor Poncelet contributed to formalizing kinetic energy as the energy due to motion, applying these concepts to the design of machines and the study of mechanical processes.
20th century and beyond: Relativity and quantum mechanics
The arrival of the 20th century brought with it revolutionary theories that expanded and challenged our understanding of kinetic energy. Albert Einstein's theory of special relativity showed that the kinetic energy of an object increases without limit as its speed approaches that of light, a conclusion that contrasts with the predictions of classical mechanics.
Simultaneously, the development of quantum mechanics introduced concepts of kinetic energy on atomic and subatomic scales, where particles can behave as both waves and particles. This led to new ways of understanding kinetic energy in the realm of the very small, where the laws of classical physics no longer apply in the same way.
Problems and solved exercises on kinetic energy
This section is dedicated to exploring practical examples and worked out exercises related to kinetic energy, presented in an accessible way without the need for complex mathematical formulas. Our objective is to illustrate how the concept of kinetic energy is applied in everyday situations and in specific problems, facilitating a more intuitive understanding of this phenomenon.
Example 1: The Cyclist and the Slope
Problem: Consider a cyclist who begins to descend a slope without pedaling. As he descends, his speed increases. How does this affect his kinetic energy?
Simplified Solution: As the rider descends, his speed increases due to gravity pulling him down. Without using formulas, we can understand that his kinetic energy also increases. This is because the faster you move, the more movement energy you have.
Example 2: Braking of a Car
Problem: A car is traveling at high speed and suddenly brakes to a stop. What happens to the kinetic energy of the car?
Simplified Solution: When the car brakes, its speed decreases until it comes to a complete stop. This means that its kinetic energy, which is the energy it had due to its motion, is reduced until it has no more, because the car is no longer moving. Kinetic energy has become another form of energy, such as the heat generated by brake friction.
Example 3: Ball in Free Fall
Problem: Imagine a ball that is released from a certain height and falls freely towards the ground. How does its kinetic energy change as it falls?
Simplified Solution: At the beginning, when the ball just starts to fall, its speed is very low, so its kinetic energy is small. As the ball continues to fall, its speed increases due to gravity, which means its kinetic energy also increases. Just before hitting the ground, the ball would have its maximum kinetic energy.
Example 4: Skater on an Ice Rink
Problem: A skater slides on an ice rink with a constant speed. If he suddenly starts skating faster, what happens to his kinetic energy?
Simplified Solution: By increasing his speed, the skater is increasing his kinetic energy. This is because, by moving faster, the amount of movement energy it has is greater. Although we don't use formulas here, the basic principle is that kinetic energy is directly related to motion: the greater the speed, the greater the kinetic energy.